Introduction
Bullhead sharks (Heterodontiformes) represent a monophyletic group that occupies a basal position within modern sharks, rays, and skates (Neoselachii) (Carvalho 1996). Remains of Heterodontiformes are among the oldest in the fossil record for Neoselachii (Kriwet 2008). Three genera are known from the Jurassic as a result of their rapid diversification: Proheterodontus and Paracestracion, which disappear from the fossil record before the Cretaceous, and the extant Heterodontus, which underwent further radiation in the Cretaceous.
A newly discovered complete heterodontid specimen from the Kimmeridgian of Eichstätt, Germany, hereafter referred to as P. danieli sp. nov., was the subject of an analysis of meristic characters in order to inform on the past biodiversity of this group. This sub-adult specimen was compared to the holotype of Paracestracion falcifer (AS-VI-505) and to extant heterodontid species.
Dentition
The change in tooth morphology during the ontogeny of extant heterodontids is gradual, resulting in pronounced ontogenetic heterodonties. Heterodontid teeth reduce from multiple cusps in juveniles to a single cusp in adults; for example, symphysial teeth of H. japonicus reduce from seven cusps at a body length of 225 mm to a single cusp at a body length of 750 mm (Figure 1). The P. falcifer holotype exhibits anterior teeth with one cusp at a body length of 400mm; this difference is substantial if it possessed seven cusps at a body length of 225 mm as it does in P. danieli sp. nov. Thus the difference in number of cusps in anterior teeth relative to the total body length suggests that the fossils represent different species.
The number of tooth families in heterodontids increases throughout ontogeny and has a species-specific pattern due to differential growth of jaws (Reif 1976). In extant heterodontids with a similar body length of 225 mm, the Meckel’s cartilage contains 13–17 tooth families, while the palatoquadrate contains 17–21 (Reif 1976). A higher number of tooth families in P. danieli sp. nov. (21 and 23 families, respectively) indicates elongation of the jaws earlier in ontogeny than in extant heterodontids, potentially reflecting different feeding ecologies (Table 1). Additionally, extant heterodontids show an increase of one to two tooth families on the palatoquadrate from a body length of 225 mm to 400 mm (Reif 1976). Therefore, the difference in number of tooth families on the palatoquadrate between P. danieli sp. nov. (23) and the P. falcifer holotype (29) is more than expected if they were of the same species (Table 1).
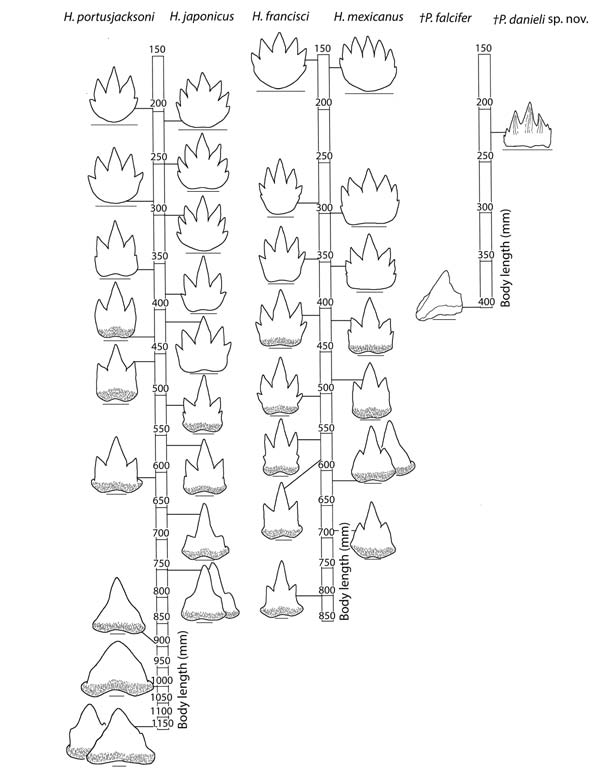
Axial body
The genus Paracestracion possesses one dorsal fin spine anterior to each of the two dorsal fins, the first associated with the pelvic fins. Spines are supported by basal cartilages, which also support fin radials (Maisey 1979). Positioning of the median and paired fins is under genetic control, and the close association of fin spines with the dorsal and pelvic fins suggests they are also involved in dorsal fin modularity (Mabee et al. 2002).
The anterior fin spine position shows no ontogenetic change in extant heterodontids, making it a reliable character in taxonomy. Sexual dimorphism is a probable explanation for the difference between P. viohli and P. falcifer; P. danieli sp. nov., however, differs from all P. falcifer specimens in the position of the anterior fin spine, which is positioned at the 9th vertebra (Table 1) (Daniel 1914). In Etmopterus spinax, the posterior fin spine position is conserved, likely due to its association with the pelvic girdle (Tave 1984). Similarly, the conserved position of the anterior fin spine in Paracestracion is potentially due to modularity with the pelvic girdle. Migration of the pelvic fins in Heterodontus might have been a result of de-coupling in this module from a common ancestor in the Lower Jurassic. The posterior fin spine position shows no consistency other than in Heterodontus, which could have resulted from genetic alterations to fin positioning.
Species name | TBL (mm) | No. tooth families | Axial body | |||||
---|---|---|---|---|---|---|---|---|
|
| Meckel’s cartilage | Palato-quadrate | 1st DFS | 2nd DFS | Pectoral girdle | Pelvic girdle | |
†Paracestracion danieli sp. nov. | 225 | 21 | 23 | 32, 33 | 62, 63 | 12 | 32 | |
*†Paracestracion falcifer | 400 | ? | 29 | 23, 24, 25 | 43, 44 | 10 | 24 | |
†Paracestracion falcifer | ? | ? | ? | 24, 25 | 52, 53 | ? | 24 | |
†Paracestracion falcifer | ? | ? | ? | 24, 25 | ~50? | 10 | 24 | |
†Paracestracion viohli | ? | ? | ? | 22, 23 | ? | ? | ? | |
Heterodontus francisci | 300 | 15 | 20 | 15, 16 | 46, 47 | 8 | 28, 29 | |
Heterodontus japonicus | 280 | 17 | 19 | 15, 16 | 46, 47 | 8 | 28, 29 |
Despite this conservation in the position of pectoral fins, the position of the coracoid and puboischiadic bar differs between the new specimen, other Paracestracion species, and extant Heterodontus (Table 1) (Mabee 2002).
Distance measurements
Seven distance measurements were taken from P. danieli sp. nov., the holotype of Paracestracion falcifer, extant juveniles of H. japonicus, H. zebra, H. philippi, and two adult H. japonicus to identify differences in body shape throughout ontogeny and between species. The Past3 software package was used to convert measurements into a ratio of length and to perform a Principal Components Analysis (PCA).
The PCA results indicate a strong divergence between P. danieli sp. nov., P. falcifer, and Heterodontus, with the highest level of variation being the distance between the pectoral and pelvic fins, and the second being the distance between the posterior dorsal and caudal fin (PC1=78.9%; PC2=15.9%) (Figure 2). The difference between P. danieli sp. nov.. and P. falcifer is greater than that seen between juvenile and adult H. japonicus, supporting the hypothesis that P. danieli sp. nov. and P. falcifer are different species.
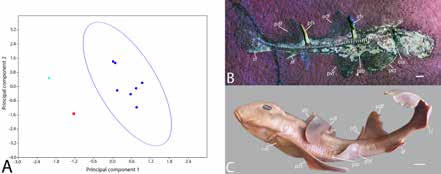
Concluding remarks
A comparison of meristic characters suggests that P. danieli sp. nov. is a separate species to that of P. falcifer and thus that extinct heterodontids were more diverse than previously thought. Future research will use cladistics approaches to investigate inter-relationships of Heterodontiformes.
Acknowledgements
I am grateful for the Palaeontological Association Undergraduate Research Bursary PA-UB201606 that financially supported this project. I would like to thank my supervisors, K. Ashbrook and J. Kriwet for their support and expertise, and P. Bartsch and J. Klapp at the Museum für Naturkunde Berlin for access and assistance with their collection.
REFERENCES
CARVALHO, M.R. 1996. Higher-level elasmobranch phylogeny, basal squaleans, and paraphyly. In STIASSNY, M.L.J., PARENTI, L.R. and JOHNSON, G.D. (Eds.) Interrelationships of fishes. Academic Press, Cambridge. pp. 35–62.
DANIEL, J.F. 1914. The anatomy of Heterodontus francisci. University of California Publications in Zoology, 13, 147–166.
KRIWET, J. 2008. A new species of extinct bullhead sharks, Paracestracion viohli sp. nov. (Neoselachii, Heterodontiformes), from the Upper Jurassic of South Germany. Acta Geologica Polonica, 58, 235–241.
MABEE, P.M., CROTWELL, P.L., BIRD, N.C. and BURKE, A.C. 2002. Evolution of median fin modules in the axial skeleton of fishes. Journal of Experimental Zoology Part B: Molecular and Developmental Evolution, 294, 77–90.
MAISEY, J.G. 1979. Finspine morphogenesis in squalid and heterodontid sharks. Zoological Journal of the Linnean Society, 66, 161–183.
MAISEY, J.G. 2012. What is an ‘elasmobranch’? The impact of palaeontology in understanding elasmobranch phylogeny and evolution. Journal of Fish Biology, 80, 918–951.
REIF, W.-E. 1976. Morphogenesis, Pattern Formation and Function of the Dentition of Heterodontus (Selachii). Zoomorphologie, 83, 1–47.
TAVE, D. 1984. Quantitative genetics of vertebrae number and position of dorsal fin spines in the velvet belly shark, Etmopterus spinax. Copeia, 3, 794–797.